
Do you have a favorite kind of weather that you love to experience? For me, it’s the first warm evening of spring, when the air is just warm enough and the wind just strong enough for the air to feel as though it is dripping off my fingers. On nights like that, I can tell that the air really is a fluid, the topic of this week’s blog.

What causes atmospheric weather patterns?
You may have noticed this year that the weather patterns across both the United States and Europe have been very persistent. That has led to the occurrence of record-setting high temperatures in some locations like the Pacific Northwest and the central US and southern Europe as well as day after day of rain in the Southeastern US. Both of these weather patterns have caused no end of grief for gardeners and farmers, since weather is seldom stuck on day after day of “perfect” conditions (even if you could define what those are).
To understand how weather patterns get stuck, it helps to know how the air moves through the atmosphere. Wind is driven by differences in heating between two areas. That contrast leads to differences in density and pressure between the areas, and the air flows from higher pressure to lower pressure to try to equalize the amount of air between those areas. The large-scale weather patterns across the globe are caused by differences in the sun heating the spherical earth at the equator and at the poles at different angles due to latitude. There are also smaller-scale wind circulations due to differences in heating between land and water (oceans or lakes) that cause the same movement of air molecules. The earth is not uniform, with continents in some areas and oceans in others and has mountain ranges that also divert the flow of air. The earth is also rotating, and that makes the wind appear to be turning towards the right in the Northern Hemisphere (left in the Southern Hemisphere), which we call the “Coriolis force.” Friction can also slow down the wind and cause it to change direction near the earth’s surface, which adds to the calculation.
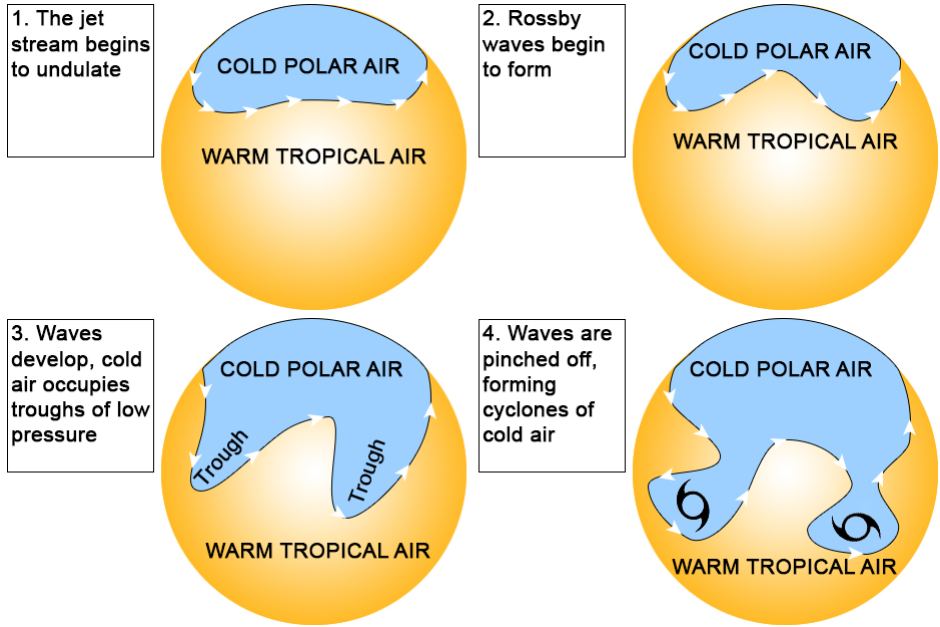
Atmospheric waves
The net result of all these forces acting on the air are a series of atmospheric waves of high and low pressure around the earth that control where the weather goes (these are known as Rossby waves after the great meteorologist Carl-Gustav Rossby). A great website to view these waves is at https://earth.nullschool.net/, with a dynamic view of the air flowing around the earth. You can move the earth around with your mouse by clicking and dragging it, and you can change the size by pinching and dragging on your touchscreen if you don’t have a mouse with a wheel. If you click on “earth” on the bottom left of their map, it pulls up a menu that allows you to pick different heights in the atmosphere (1000 mb is closest to the surface, 500 mb is about halfway up in the atmosphere, and 250 mb (shown in this figure from July 29, 2021) is roughly the height that jets fly.
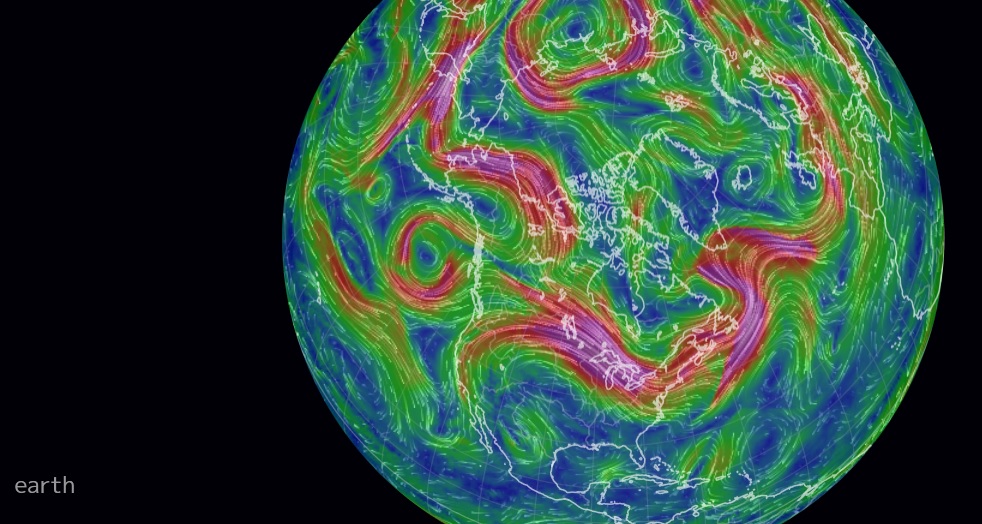
The image shows areas of strong winds (in red) and weak winds (in blue). The strong winds at 250 mb are called “jet streams”, and they push weather systems around. The strong jet stream over the upper Great Lakes in this image helps explain why the severe weather that moved through Wisconsin on July 29 moved from northwest to southeast. The jet streams outline the atmospheric waves that control the large-scale weather patterns. In the Northern Hemisphere, winds blow clockwise around areas of high pressure (“ridges”) and counterclockwise around low pressure (“troughs”). It is much easier to see on the website than on this static image, although traditional weather maps usually show the wind direction using arrows to help. As the image shows, often a ridge in one half of the continent is accompanied by a trough in the other half just like an ocean wave.
The large-scale weather patterns that are connected to atmospheric waves are constantly shifting as the air in the atmosphere tries to balance out all the forces that are pushing it around. (This 30-second video shows how the waves typically evolve over time.) Often the waves move, usually from west to east in the mid-latitudes, and so the weather at those latitudes also tends to move from west to east. But sometimes the weather patterns get stuck in one spot, and cause day after day of the same weather. We call these “blocking” patterns because they block the natural movement of the waves, locking the weather pattern in place for long periods. That is what we have seen this summer, with a very strong high-pressure center locked over the western U. S. and a trough of low pressure draped over the eastern U. S. for much of the last few months. In summer, high pressure usually causes sinking air, lack of clouds, warm temperatures, light winds, and no rain. In contrast, low pressure leads to rising air that forms clouds and rain and cooler temperatures due to the clouds.
What is happening this summer?
The blocking this summer has been more persistent than usual, with strong high pressure in the west causing a very hot and dry area to form (sometimes called a “heat dome”). Once you get a strong high like that to form, it can get anchored in place by the dry conditions and high temperatures at the surface, making it very hard to move. The length of time that this summer’s high has lasted has contributed to the string of record-setting temperatures and lack of rain and subsequent drought that region has experienced this year. By contrast, in the Southeast, low pressure has led to a stubborn low-pressure trough that has brought rain to the region almost every day, preventing farmers from doing field work or drying hay and increasing the occurrence of fungal diseases on their crops. If you know you are in a blocking pattern, that means you should prepare to experience the same weather for protracted periods of time and adjust your gardening schedule to accommodate the weather you are likely to see for the next week.
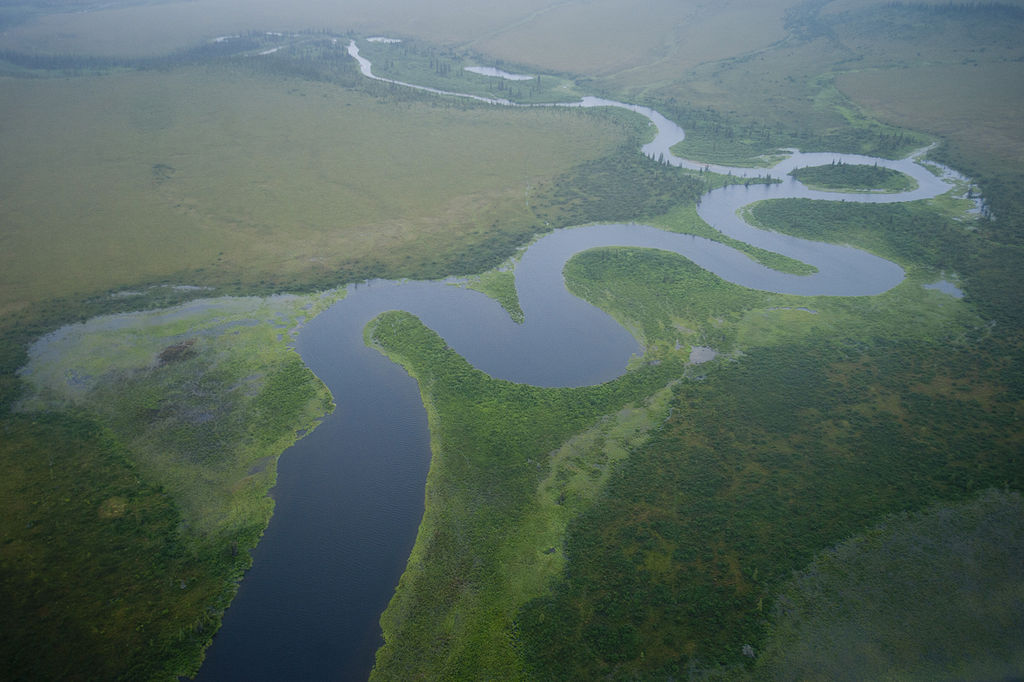
How will atmospheric patterns change in the future?
Some but not all atmospheric scientists think that as the earth gets warmer, the temperature difference between the equator and the poles will decrease and that this will lead to atmospheric wave patterns that are loopier, like winding rivers in an almost flat coastal plain. This could lead to both more extreme weather and potentially, more frequent blocking patterns. Gardeners need to prepare by designing their gardens to handle both more extremes of weather, including heat waves and floods, and periods of more persistent weather, which could lead to more frequent and longer droughts. That means making sure that you need to have good drainage for high-intensity rainfall events but also need to use methods like mulching with arborist chips to preserve the moisture in the soil for those times when no rain is in the forecast for extended periods.
